"LDL may or may not correlate to cardiovascular
outcomes,”
- Dr. Kim Allan
Williams, president of the American College of Cardiologists
“God, grant me the serenity to accept the things I cannot
change,
The courage to change the things I can,
And the wisdom to know the difference.”
- Reinhold Niebuhr’s Serenity Prayer
Last year there was a good discussion of the saturated fat
issue on Otago University's Public Health Expert blog (here) that continued
into the comments.
David Brown pointed out that
"Evaluation of the overall health effects of saturated
fat requires consideration of markers in addition to LDL-cholesterol.
Isocaloric replacement of carbohydrate with any type of fat results in
decreased triglycerides and increased HDL-cholesterol, the effect on
HDL-cholesterol being greater for saturated fat compared to unsaturated fat.
Reductions in saturated fat also adversely affect HDL subpopulations by
decreasing larger HDL2-cholesterol concentrations, whereas increases in
saturated fat increase this antiatherogenic fraction. "
In response to this, Tony Blakely, professor of public
health, commented
"A note of caution on HDL. An important and massive
analysis of many studies published in the Lancet in 2012 found no association
of HDL with myocardial infarction (or heart attacks). This study used a genetic
technique call Mendelian Randomisation, which strips away (in theory, and I
believe in practice in this paper) all the confounding that plagues
observational studies. Thus, it appears that it is LDL – not HDL – that has a
causal association with coronary heart disease.
Reference: Voight BF, Peloso GM, Orho-Melander M, et al.
Plasma HDL cholesterol and risk of myocardial infarction: a mendelian
randomisation study. The Lancet 2012;380(9841):572-80 doi:
10.1016/s0140-6736(12)60312-2[published Online First: Epub Date]|."
At the time I thought fine, when the evidence runs against
your hypothesis, invent a new statistical method that obscures the fact.
Nothing to see here. Then a few weeks ago Bill Barendse tweeted a paper that
used Mendelian Randomisation and Bill to put it mildly has expertise in the field
of genetics, so I thought it might not hurt to look into this again.
Though I am doing so in a superficial way, using logic
rather than any deep understanding of the genome, I want to question whether
the idea that HDL is not causal in CVD, if it is true, should make any
difference at all to how we interpret risk factors or the effect of dietary or
lifestyle changes.
Mendelian Randomisation is a method for identifying drug
targets. If people with X polymorphism have the same cardiovascular risk as
everyone else, then there's not much point in developing a drug that targets X.
Fair enough - drugs that elevate HDL by tweaking the enzymes associated with it
have been a big disappointment.
The problem is, the cardioprotective association of HDL
remains for all that. And, as for "all the confounding that plagues
observational studies", HDL is measured directly, whereas LDL is
calculated in diverse ways; and it's hard to see how confounding applies to
blood tests in the sense that it applies to diet epidemiology. The examples
here involve controlled conditions and short-term hard outcomes in secondary
prevention. This is as good as it gets - high HDL is indeed a solid marker for
cardioprotection and lots of other good things (albeit the HDL increase in response to alcohol probably weakens this in some populations). Does it matter whether the HDL particle itself is protective in a causal fashion? (and, if the particle that removes cholesterol from foam cells is really a dud, where does that leave the lipid hypothesis?)
Should we rely on LDL alone to assess cardiovascular risk?
(TG isn't convincingly associated with risk in Mendelian Randomisation either)
Well, not if we have those other CVD risk factors, insulin resistance or type 2
diabetes.
"When compared with IS, the IR and diabetes subgroups
exhibited a two- to threefold increase in large VLDL particle concentrations
(no change in medium or small VLDL), which produced an increase in serum
triglycerides; a decrease in LDL size as a result of an increase in small and a
reduction in large LDL subclasses, plus an increase in overall LDL particle
concentration, which together led to no difference (IS versus IR) or a minimal
difference (IS versus diabetes) in LDL cholesterol; and a decrease in large
cardioprotective HDL combined with an increase in the small HDL subclass such
that there was no net significant difference in HDL cholesterol. We conclude
that 1) insulin resistance had profound effects on lipoprotein size and
subclass particle concentrations for VLDL, LDL, and HDL when measured by NMR;
2) in type 2 diabetes, the lipoprotein subclass alterations are moderately
exacerbated but can be attributed primarily to the underlying insulin
resistance; and 3) these insulin resistance-induced changes in the NMR lipoprotein
subclass profile predictably increase risk of cardiovascular disease but were
not fully apparent in the conventional lipid panel."
("LDL may or may not correlate to cardiovascular
outcomes")
Garvey WT et al. Effects of insulin resistance and type 2
diabetes on lipoprotein subclass particle size and concentration determined by
nuclear magnetic resonance. Diabetes. 2003 Feb;52(2):453-62.
Are there other interpretations of Mendelian Randomisation
in the literature?
"Observed associations between serum CRP and insulin
resistance, glycemia, and diabetes are likely to be noncausal. Inflammation may
play a causal role via upstream effectors rather than the downstream marker
CRP."
Brunner EJ et al. Inflammation, Insulin Resistance, and
Diabetes—Mendelian Randomization Using CRP Haplotypes Points Upstream. Plos
Medicine August 12, 2008 DOI: 10.1371/journal.pmed.0050155
In other words, those markers that don’t have genetic links
to the incidence of a condition should be considered as downstream effects of
the true cause. A drug that blocks CRP synthesis won’t prevent diabetes (or any
other inflammatory condition) – why should it? Most likely CRP is just doing
its job, and things would not suddenly be brilliant if it was removed.
Let’s walk this idea back to lipids and CVD. The TG/HDL ratio isn’t determined
by your lipid genes, it’s a downstream effect of dietary carbohydrate
(non-genetic) and insulin resistance (genes linked to IR and hyperglycaemia do
correspond to CVD). The association between LDL and cardiovascular risk is
modified by carbohydrate which increases TG-rich VLDL, the end product of which
is the small, dense LDL particle, which is cleared more slowly than larger LDL
particles and is thus exposed to peroxidation. Another effect of having a high
output of TG-rich VLDL being that HDL gets loaded with TGs and is cleared from
circulation faster (hence high TG, low HDL). Half of your LDL-associated risk
can be traced to genes (like ApoE4) which you can try to tweak with drugs if
you like, and half belongs to Beta-apolipoprotein, sdLDL, oxLDL etc, which are
modified by the carbohydrate factor. Saturated fat effects on VLDL and LDL may
differ depending on the foods they are in or the other macronutrients ,
especially carbohydrate.
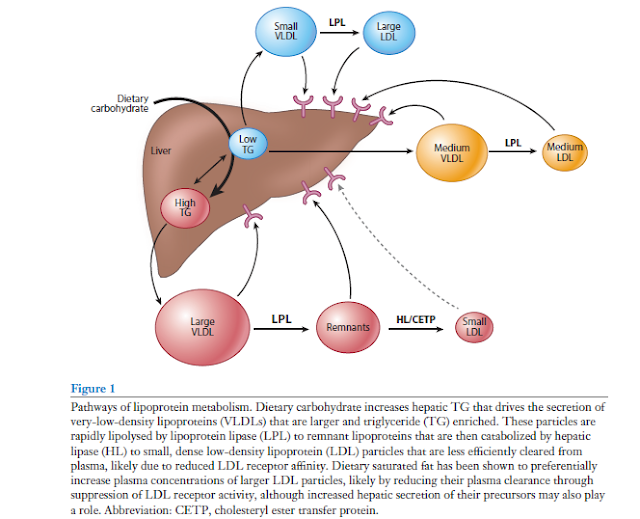 |
From Siri-Tarino et al 2015 |
Your liver is downstream from your gut and has first pass at the nutrients you
absorb there; its uptake of fats, sugars and proteins determines the
triglycerides, cholesteryl esters, and apolipoptoteins the liver produces and
its types of HDL and LDL species. Genetics has more influence on the LDL
species than on the HDL or TG, and if you are insulin-resistant the effect of high-carbohydrate
diet on HDL, TG-rich VLDL, and atherogenic LDL subspecies is magnified; this is the pathology
that hyperlipidaemia, MetSyn, and diabetic lipid patterns have in common.
This is how Mendelian Randomization of LDL and HDL was presented in a recent
butter and cholesterol paper [here]
“The LDL-cholesterol concentration is a true risk factor for CVD. A
meta-analysis of 26 trials showed that, for every 1-mmol/L reduction in LDL
cholesterol, there was a 20% relative reduction in deaths that were due to
coronary heart disease (RR: 0.80; 99% CI: 0.74, 0.87) (30). Thus, our result of
an increase in LDL-cholesterol concentration of 0.16 mmol/L was not negligible.
In addition, butter resulted in a concomitant increase in HDL cholesterol
compared with the habitual diet. An increase in HDL cholesterol of the butter
diet rich in long-chain SFAs was expected because these SFAs are known to
increase HDL cholesterol…
According to the literature, the HDL
cholesterol concentration is associated with a protective effect on CVD (31,
32). However, studies that used Mendelian randomization showed that genetically
decreased HDL cholesterol did not increase risk of myocardial infarction and
questioned a causal association between the HDL concentration and CVD (33–35).
Thus, it is necessary to be careful with interpreting low HDL-cholesterol
concentrations as a CVD risk factor. However, as a marker of cardiovascular health, changes in HDL cholesterol
concentrations need to be included when interpreting the effect of SFAs in the
diet. It is possible to speculate that an unbeneficial increase in LDL
cholesterol may partly be counteracted by the beneficial effect of SFAs on HDL
cholesterol, which suggests that dairy and saturated fat may be less harmful in
relation to CVD than previously thought, as reported in recent meta-analysis
(8, 9).”Engel S and Thorstrup T (2015) Butter increased total and LDL cholesterol compared with olive oil however resulted in higher HDL cholesterol than habitual diet. Am J Clin Nutr. ajcn112227
Another suggestion is that HDL functionality is the important variable. HDL
functionality is increased by CLA in butter and ruminant fat, olive oil polyphenols,[1] and the action of vitamin E (found in nuts and vegetable oils and other sources
of linoleic acid) on protein kinase-C.[2] As substitution of all fats for
carbohydrates tends to raise HDL, there will be a correspondence between intake
of natural fats, HDL, and HDL functionality. Polyphenols administered without
fat reduce inflammation but do not increase HDL or HDL functionality.[3]
Thus there is evidence for a rather neat correspondence between the quality of
dietary fat and the cardioprotection associated with HDL -
[1] Hernáez Á et al (2014) Olive oil polyphenols enhance
high-density lipoprotein function in humans: a randomized controlled trial. Arterioscler
Thromb Vasc Biol. 2014 Sep;34(9):2115-9. doi: 10.1161/ATVBAHA.114.303374. Epub
2014 Jul 24.[2] Mendez AJ et al (1990) Protein Kinase C as a Mediator of High Density Lipoprotein Receptor dependent Efflux of Intracellular Cholesterol (1990) Journal of Biological Chemistry Vol. 266, No. 16, Issue of June 5, pp. 10104-10111,199
[3] Nicod N et al (2014) Green tea, cocoa, and red wine
polyphenols moderately modulate intestinal inflammation and do not increase
high-density lipoprotein (HDL) production. J Agric Food Chem. 2014 Mar
12;62(10):2228-32. doi: 10.1021/jf500348u. Epub 2014 Mar 4.
From a 2014 paper that failed to find a causal relationship between HDL and CVD:
The estimates of LDL-C from instrumental variable analysis showed that a long-term genetically increased LDL-C, regardless of the analytical strategy used (unrestricted, restricted, or unrestricted score plus sequential adjustments) resulted in an increased causal OR for CHD, which is similar in magnitude to that reported in randomized trials of statin-lowering therapies in individuals at low risk of vascular disease1 and is further evidence of the validity of our various analytical approaches.For triglycerides, the findings for the unrestricted and restricted allele scores were concordant, with both showing association with CHD. However, the unrestricted score adjusted for HDL-C diminished the association to null.This could mean that a treatment that targets a triglyceride pathway that has no effect on HDL-C may not be beneficial, whereas a treatment that targets a triglyceride pathway that both reduces triglycerides and increases HDL-C could have a role in prevention of CHD events. An alternative explanation is that HDL-C could mark long-term triglyceride concentrations, but this hypothesis requires further investigation.
Holmes,
MV, et al. Mendelian randomization of blood lipids for coronary heart disease. 2014. DOI: http://dx.doi.org/10.1093/eurheartj/eht571
Clearly, the complete implications of mendelian randomization for
cardiovascular risk related to diet are far from clear.
But I'd putting money on this; these analyses don’t change the meanings of metabolic risk factors that are affected
by diet and lifestyle, and if anything they support their usefulness as measures of improvement.